The desert sultanate of Oman is home to some of the weirdest—and possibly most useful—rocks on earth. The stark Hajar mountains, near the border with Saudi Arabia, contain a chunk of earth’s mantle—a zone that makes up most of earth’s mass, but normally lies inaccessible to humans, far below the surface. Here, though, a sliver of mantle has made its way up to where we can see and touch it. The outcrop has drawn scientists looking for clues to the dynamics of the deep earth; the origins of life; and, most recently, ways to fight climate change.
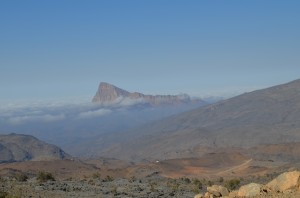
Peter Kelemen, a geologist at Columbia University’s Lamont-Doherty Earth Observatory, is spearheading an international group that one day hopes to drill into the formation to examine such questions. The thrust of his own research is the prospect of speeding up recently documented chemical reactions within the rocks that he thinks could draw large amounts of globe-warming CO2 from the atmosphere and entomb it back in the earth.
The formation, called the Samail ophiolite, comprises the remains of an ancient seafloor. It covers an area the size of Massachusetts five kilometers thick—the world’s largest such exposure. Smaller bits of mantle rocks are found along the coasts of Cyprus, Papua New Guinea, Newfoundland, Costa Rica and northern California. The most common mantle rock, known as peridotite, is loaded with exotic gemlike minerals and is characteristically smooth and greenish—hence “ophiolite,” from the Greek ophis (snake) and lithos (rock).
Many scientists think the Hajars appeared 70 million to 95 million years ago, when normal tectonic processes went awry. As plates making up earth’s quiltlike surface push against each other, usually plates of seafloor crust (made of heavy volcanic rock) dive below plates of land (made of lighter rock) and get subsumed. Here, though, a chunk of seafloor—and some of the mantle undergirding it—somehow got plowed up onto dry land and piled up to form the mountains. In many places here, the sediments and crust once forming the seabed have subsequently eroded, exposing underlying mantle rocks that once lay some 15 kilometers below the seabottom. (Under continents, the mantle generally lies much deeper–often 75 kilometers.)
The elements and compounds in the mantle are distinct from those of the surface, putting the two domains out equilibrium; that is, like old friends who haven’t seen each other for many years, they have a lot to talk about, and once they meet, they throw themselves into a kind of chemical conversation, reacting rapidly with each other. One key reaction: mantle rocks react rapidly with the carbon dioxide that is abundant in air and near-surface groundwater, but which is unavailable in the deep earth. The reaction turns the CO2 into a carbonate—a solid limestone-like mineral that locks the CO2 away essentially forever. The results can be seen throughout the Samail ophiolite, in whitish carbonate veins lacing outcrops, stalactites dripping off cliffs, and powdery crusts precipitating out of springs and streams.
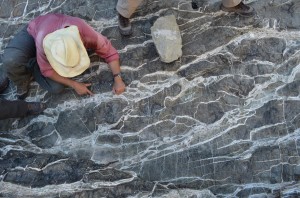
Geologists have long recognized that these processes take place. But recent studies by Kelemen and colleagues have revealed that the reactions are far more rapid and prevalent than previously thought. Scientists had assumed that most visible carbonates formed long ago. However, by sampling rocks from road cuts, wells, boreholes and other sources, Kelemen and his partner geochemist Juerg Matter (a Lamont adjunct, now based at the University of Southampton) have found that many carbonate veins here actually formed during human time, and that the process is ongoing and fast. “It happens about 100,000 times faster than we thought,” said Kelemen. They also found that the reactions take place much deeper underground than previously thought, well below the surface where CO2 travels in groundwater through cracks.
Some underground reactions are so vigorous, they appear to create small explosions—a sort of natural hydrofracking–that open more cracks, creating space for more reactions, and thus feeding a continuous loop of CO2 uptake. Kelemen and Matter estimate that each cubic kilometer of peridotite naturally absorbs on average a ton of atmospheric carbon each year–up to 100,000 tons annually in the region. In places, the reaction has completely run its course; the rocks are fractured down to the tiniest pore spaces and filled with carbonate, creating entire mountains of the stuff. Theoretically, they say, there is enough peridotite in Oman and the neighboring United Arab Emirates to absorb 33 trillion tons of CO2—1,000 years of human output, if present-day emission rates remained unchanged.
“I’m one of the 98 percent of scientists who has looked at this and believes CO2 is a greenhouse gas,” said Kelemen. “Every single calculation you can do will tell you if you increase CO2 in the atmosphere, the earth is going to warm up. That extra energy is going to move the weather around in unpredictable ways and bring big changes in climate. And almost surely, because there are now so many people inhabiting the surface of the planet, we’re going to find that those most of those changes are negative.”
With CO2 concentrations recently measured at 400 parts per million probably for the first time in some 3 million years, and little movement toward reducing industrial emissions, many scientists are now looking at ways to remove the gas from the air.
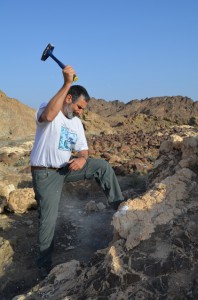
No one thinks that Oman’s rocks could solve the whole problem. But with some simple engineering, Kelemen and Matter think natural process could be speeded up a million times in places, and that could take up some of manmade CO2. One way would be to drill boreholes and pump down heated water impregnated with CO2; heat greatly speeds the reaction, and once jump-started this way, the cycle of natural cracking would do the rest, rapidly taking in any more CO2 pumped down. Oman is rich in oil and burns a lot of it in power plants, so much CO2 could be delivered from the plants in pipelines. Another
method would take CO2 directly from the air. This would involve pumping surface seawater, which naturally absorbs atmospheric CO2, down pipes into the mantle rocks just offshore. The cleaned-up water would then be flushed back to the sea surface, where it would then absorb more CO2. Accounting for engineering challenges and the obviously large expense, the researchers assert that Oman’s peridotite still might be harnessed to absorb as much 4 billion of the 30 billion tons of the atmospheric CO2 currently produced annually by humans.
In 2012, experts from 11 countries held a workshop to discuss drilling experimental boreholes into the ophiolite. This would not only advance the carbon-storage idea, but help researchers understand other questions. These include how the crust and mantle interact to fuel volcanism; and how reactions with the mantle might contribute to formation of metal deposits, such as Oman’s own valuable mines of copper and chrome. The mantle-crust transition zone also produces methane and other substances that evolutionary biologists think may have incubated the first microbes on earth. Today, tiny subsurface creatures persist, but they are poorly known; further exploration of the ophiolite might shed light on them, and the history of life on earth itself.
For now, Kelemen and a few students are doing the basic work on foot, scrambling over the rough terrain to map the rocks, and performing lab experiments. “By hiking down a beautiful canyon in Oman, we can basically walk 20 kilometers into the earth’s interior and see how it works,” said Kelemen. That said, given current political and economic realities, he is skeptical that any carbon-storage scheme will come to quick fruition. “I’m not convinced that society is really going to do very much about [climate change] until we find out what the long-term effects are going to be,” he said. “But at that time, there might be renewed interest in how you might get CO2 out of the air. We’re trying to develop methods so that when and if we do get ready to use them, they’ll be available.”
RELATED:
Carbon-Capturing Rocks, Technology Review
Reactive Rocks, Nature Climate Change